Antiprotons from Beyond the Solar System
N. Eugene Engelbrecht and R. Du Toit Strauss
Centre for Space Research, North-West University, Potchefstroom, South Africa
• Physics 18, 19
The spectrum of cosmic-ray antiprotons has been measured for a full solar cycle, which may allow a better understanding of the sources and transport mechanisms of these high-energy particles.
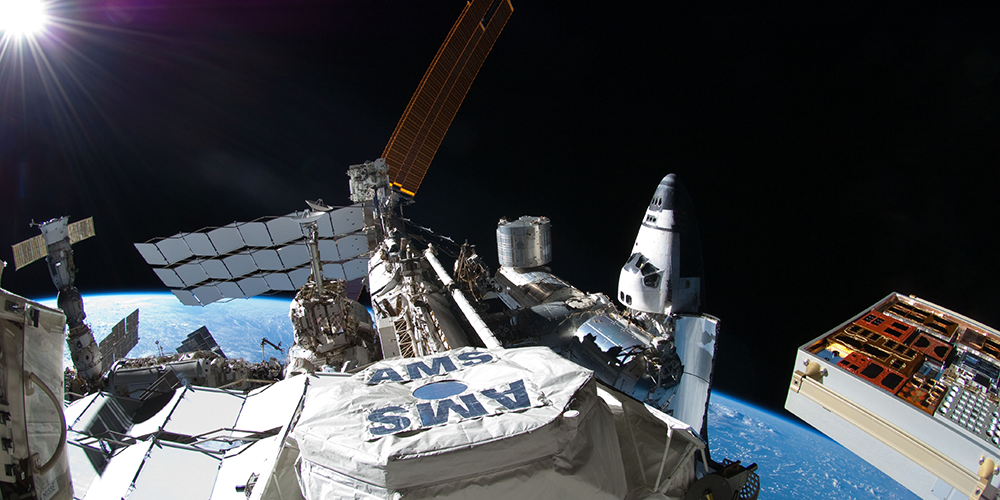
The heliosphere is a region of space extending approximately 122 astronomical units (au) from the Sun (1 au being the average distance between the Sun and Earth). This volume mostly contains plasma originating from the Sun but also various charged particles with higher energies. These particles can be categorized according to their energies and origins: Lower-energy solar energetic particles, for instance, come from the Sun itself, while Jovian electrons have their origin in the magnetosphere of Jupiter. Another such population comes from outside the Solar System: galactic cosmic rays (GCRs), which mostly consist of protons and electrons and their antiparticles and span a vast range of energies from mega-electron-volts to exa-electron-volts [1]. Astonishingly, energies at the high end of this range would correspond to a single particle carrying as much kinetic energy as a well-thrown baseball. The team operating the Alpha Magnetic Spectrometer (AMS)—a particle detector on the International Space Station (Fig. 1)—has now completed a long-term measurement of a subset of this GCR population that has been relatively uncharted until now, namely, GCR antiprotons [2]. This result will help researchers to understand the particle-transport processes that affect the GCR spectrum within the heliosphere and possibly identify the origin of these highly energetic particles.
GCRs are accelerated to their high energies through various astrophysical processes. The particles’ most likely sources within our Galaxy are supernova remnants [3], although the highest-energy GCRs are expected to be of extragalactic origin [4]. Detailed measurements of GCR intensities can, therefore, provide valuable insights into fundamental physics in distant cosmic regions. They might also provide glimpses of new physics. For instance, some GCR antiprotons could possibly be formed because of dark matter annihilation [5], the hallmark of which would be increased intensities of the GCR antiproton flux at specific energies.
These signals are difficult to pick out because of the fact that GCR particles experience several transport processes as they traverse the heliosphere. For example, they can be scattered because of turbulent fluctuations of the heliospheric magnetic field (HMF), which cause the particles to propagate diffusively. The HMF also causes GCR particles to experience drifts that depend on their charge. To further complicate matters, the strength of the HMF rises and falls with the Sun’s 11-year activity cycle, while the polarity of the HMF reverses on a 22-year cycle. During periods of positive HMF polarity, when the HMF in the northern heliosphere is pointed away from the Sun (and toward it in the southern hemisphere), positively charged GCRs drift toward the Sun from the polar regions of the heliosphere and away from it along the heliospheric current sheet (the region separating hemispheres of opposite magnetic polarity). During periods of negative polarity, the HMF direction, and hence the drift directions for positively charged GCRs, are reversed. Negatively charged GCRs, like electrons and antiprotons, drift in the opposite direction to positively charged GCRs like protons and positrons for a given polarity cycle.
Diffusion, drift, and other transport effects combine to reduce the intensity of GCRs so that the local interstellar spectrum of these particles (which could contain signals of, for example, dark matter annihilation) is modulated into the intensity spectrum observed close to Earth. Researchers have observed the time- and charge-dependent modulation of GCRs since the 1950s by detecting secondary neutrons created when cosmic rays interact with Earth’s atmosphere [6]. As a function of the 11-year solar cycle, the GCR intensity peaks during solar minima and dips during solar maxima—an effect that is especially pronounced for lower-energy particles (Fig. 2). These peaks in intensity during solar minima also reflect the 22-year magnetic-polarity cycle: A strong intensity peak is followed by a weaker one, which, in turn, is followed by another strong one, and so on. An understanding of these heliospheric modulation effects is essential for researchers to gain insights into new physics from GCR observations and can only be achieved through careful numerical modeling [7] and detailed comparison of such model results with accurate observations of multiple species of GCR.
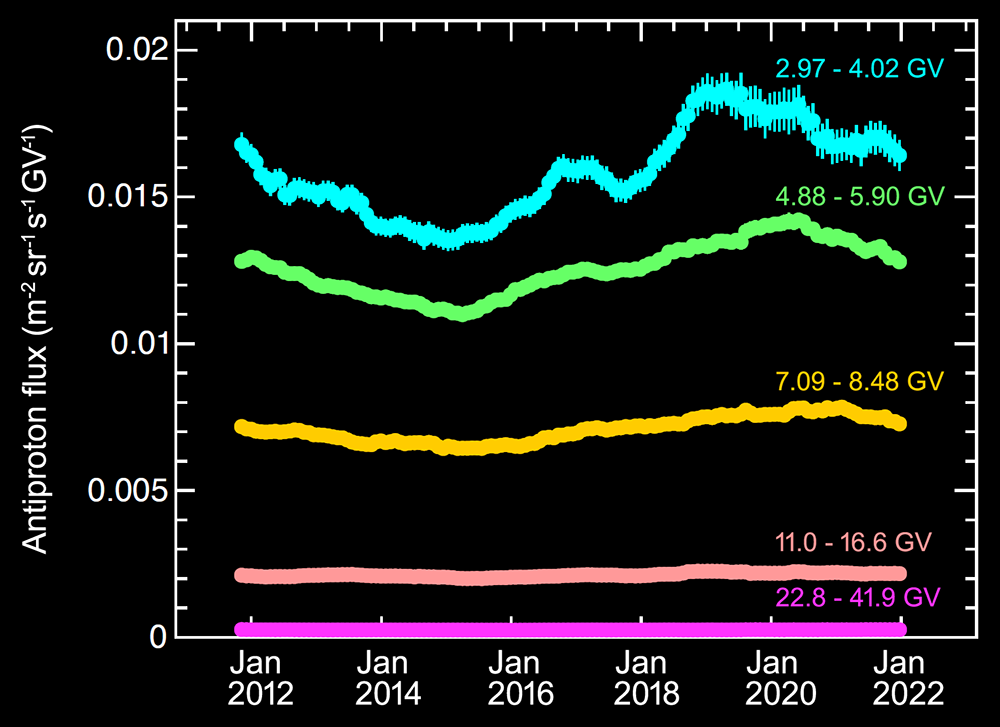
The AMS Collaboration has added an important new component to these observations by revealing antiproton intensities averaged over 27-day periods across a full 11-year solar cycle (Fig. 2) [2]. This is a considerable advance because, given the scarcity of GCR antiprotons relative to GCR protons, prior studies have only been able to obtain antiproton-intensity spectra averaged over several years of observation [8]. Furthermore, these new observations are presented in conjunction with simultaneous observations of the intensities of the other GCR components—protons, electrons, and positrons. This data combination allows direct comparisons to be made between the time- and energy-dependent intensity profiles of particles and their antiparticles. The results show a temporal antiproton intensity profile qualitatively similar to that expected from GCR modulation studies [9], with drift and modulation effects becoming less significant at higher particle energies. Such information will allow researchers to perform quantitative comparisons between model predictions and spacecraft observations, potentially yielding valuable insights into the transport processes these particles experience. Simultaneously modeling the intensities of oppositely charged protons and antiprotons would, for instance, allow detailed studies of the interplay between the diffusion of GCRs in the heliosphere—a mechanism that predominates during solar maximum—and drift effects—which play a larger role during solar minimum. Comparative studies can also now be made of the coefficients governing the diffusion of these different GCR particle species, greatly benefiting the particle-transport community. A deeper understanding of how transport processes modulate the GCR spectrum will also go a long way toward allowing researchers to disentangle solar-induced modulation effects from other contributions to the acquired spectra. This separation may assist in the search for subtle signatures of new physics, including effects related to dark matter.
References
- D. J. Bird et al., “Detection of a cosmic ray with measured energy well beyond the expected spectral cutoff due to cosmic microwave radiation,” Astrophys. J. 441, 144 (1995).
- M. Aguilar et al. (AMS Collaboration), “Antiprotons and elementary particles over a solar cycle: Results from the Alpha Magnetic Spectrometer,” Phys. Rev. Lett. 134, 051002 (2025).
- P. Blasi, “The origin of galactic cosmic rays,” Astron. Astrophys. Rev. 21, 70 (2013).
- J. R. Hörandel, “The origin of galactic cosmic rays,” Nucl. Instrum. Methods Phys. Res., Sect. A 588, 181 (2008).
- J. Heisig, “Cosmic-ray antiprotons in the AMS-02 era: A sensitive probe of dark matter,” Mod. Phys. Lett. A 36, 2130003 (2020).
- K. D. Moloto et al., “The Southern African neutron monitor program: A regional network to study global cosmic ray modulation,” Adv. Space Res. 72, 830 (2023).
- N. E. Engelbrecht et al., “Theory of cosmic ray transport in the heliosphere,” Space Sci. Rev. 218, 33 (2022).
- O. Adriani et al., “Measurement of the flux of primary cosmic ray antiprotons with energies of 60 MeV to 350 GeV in the PAMELA experiment,” JEPT Lett. 96, 621 (2013).
- N. E. Engelbrecht and K. D. Moloto, “An ab initio approach to antiproton modulation in the inner heliosphere,” Astrophys. J. 908, 167 (2021).
About the Authors
N. Eugene Engelbrecht is currently a professor in physics at North-West University, Potchefstroom, South Africa, where he received his PhD in physics. His research interests include modeling the transport of cosmic rays throughout the heliosphere from first principles, turbulence and its transport, and the diffusion of charged particles in turbulent plasmas.
R. Du Toit Strauss received his PhD in physics from North-West University, Potchefstroom, South Africa, where he is currently a professor in physics. He is an alumnus of the Fulbright and Alexander von Humboldt associations and holds an adjunct position at the Department of Space Science at the University of Alabama in Huntsville. His primary research interests involve the modeling of cosmic-ray propagation through the turbulent heliosphere.